The Kupiec laboratory uses “the awesome power of yeast genetics” to investigate basic universal processes that are very hard to study in other organisms. Our basic methodology involves Molecular Biology and Genetic techniques. But we are not driven by any particular technique. Rather, we would like to answer some very basic questions about how genomes function.
As yeast is today the best understood eukaryotic organism, with more than half of its genes with a known function/activity, the new genetic and molecular tools developed in yeast have jump-started a revolution in biology: Systems Biology. We are able, for the first time, to ask very basic questions about the way genomes are organized, genes interact, proteins talk to each other, etc. This genome-wide approach requires novel tools, which we are helping to develop in cooperation with people from Computer Science at TAU. Most of the essential pathways, complexes and genes involved in basic cellular processes are conserved in evolution, and human orthologs are present for most of the genes we study.
​
Here are some of the basic biological questions that we are trying to understand, using the baker’s yeast (Saccharomyces cerevisiae) as a model organism:

DNA repair
​
Our cells are constantly exposed to radiation and chemicals that cause damage to the DNA or even break the chromosomes in pieces. Even natural cellular metabolism creates oxidative stress and DNA damage. Luckily we have efficient mechanisms to repair the damage.
DNA repair
​
Our cells are constantly exposed to radiation and chemicals that cause damage to the DNA or even break the chromosomes in pieces. Even natural cellular metabolism creates oxidative stress and DNA damage. Luckily we have efficient mechanisms to repair the damage. But HOW do you repair a stalled replication fork or a broken chromosome? We would really like to know how this works...
Stability of the eukaryotic genome
​
Normal cells have remarkably stable karyotypes. You can easily identify to what species a cell belongs, just by looking at its chromosomes. However, cancer cells lose this stability, and start accumulating translocations, deletions, amplifications, etc. Many of the endpoints of these rearrangements fall in repeated sequences (sometimes called “junk DNA”) that fill-up our genomes. What prevents a high level of chromosomal aberrations as a consequence of recombination between repeated sequences? What mechanisms maintain the stability of the genome? We would really like to know how they work...
Telomeres​
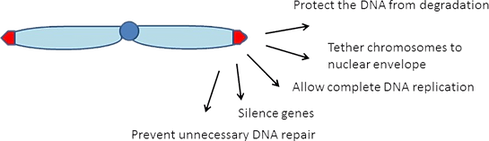
​Telomeres are nucleoprotein complexes at the end of the eukaryotic chromosomes. They are important in the development of cancer and in aging. We would like to know how do all these genes work together to regulate telomere length. Are there several pathways? Complexes? What are the interactions between the various elements? To answer these questions we are using a combination of Molecular Biology, Systems Biology, Genetics and Biochemistry. Bioinformatic models are used as a basis to plan possible experiments. The results are then incorporated into the model, to generate more predictions in a continuous cycle that progressively refines the model. How do cells know when a telomere has the right size and when it is too short? We would really like to know how this works...
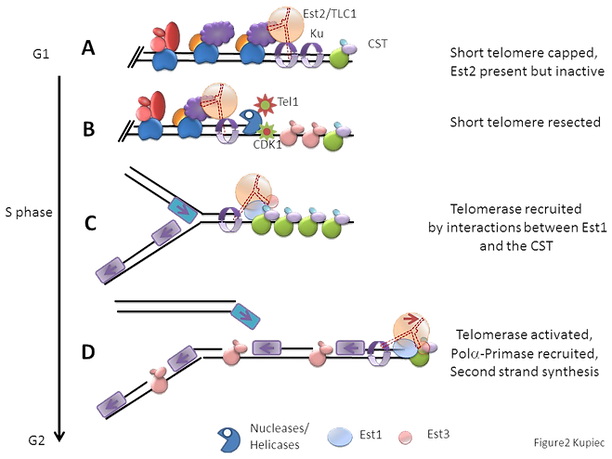
Clamp loaders and unloaders
​
During DNA replication and repair, DNA polymerases are held on the DNA by a ring or clamp called PCNA. PCNA must be loaded at the leading strand and at each of the Okazaki fragments of the lagging strand. The complex that loads PCNA is called Replication Factor C (RFC) and is composed of a large subunit (Rfc1) and 4 small subunits (Rfc2-5). We have found 3 RFC-like complexes (RLCs), all composed of the 4 small Rfc subunits, but each having a different large subunit: Elg1, Ctf18 and Rad24. These conserved complexes play central roles in DNA replication and the response to DNA damage. They are evolutionarily conserved and its human homologs are tumor-suppressing genes implicated in cancer. We would really like to know how they work...
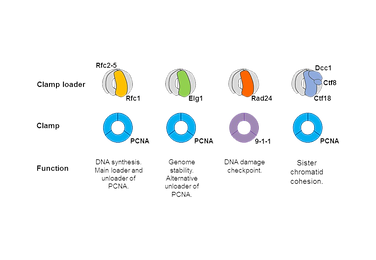

Chromatin and genome stability
​
The DNA in the nucleus is wrapped around nucleosomes, composed of histones. Chemical modifications of these proteins determine the level of accessibility of a chromosomal region to different enzymes, including those in charge of transcription. Whether a gene is expressed or silenced is transmitted from one generation to the next, as an epigenetic phenomenon. The precise mechanism that allows copying the epigenetic state each time a cell duplicates its genome is not known. We are investigating the role of DNA replication factors in this process.
Similarly, each time a genome is duplicated, twice-as-many histones are required to wrap both genomes. How do the cells coordinate DNA replication with histone deposition and histone modifications? We would really like to know how this works...

DNA replication and sister chromatid cohesion (SCC)
​​
During DNA replication each chromosome is duplicated. However, the two sister chromatids are kept together with the help of a protein complex called cohesin, until anaphase, when the two sisters are pulled to opposite poles by the mitotic spindle. How is SCC established? And what is the role of DNA replication and repair genes in this process? We would really like to know how this works...
DNA repair and cellular metabolism
​​
Cells must deal with DNA damage all the time. Some of it comes from environmental sources (radiation, chemicals), and part is due to the natural cellular metabolism. Cells must balance their metabolic needs with their response to external insults. How does metabolic state affect the response to DNA damage? This is of high medical importance, as cancer cells, as yeast cells, have special metabolic tricks (e.g.: the Warburg effect). We would really like to know how this works...
​
TOR signaling
(In collaboration with Prof. Ronit Weisman from the Open University of Israel)
​
The TOR protein kinases exhibit a conserved role in regulating cellular growth and proliferation. We use the fission yeast S. pombe to answer the following questions: What is the function of each of the two highly conserved TOR
complexes? How are they regulated? How do the TOR proteins integrate signals from the environment to know when to grow? How do they coordinate growth with the regulation of cell divisions, development, genomic and
epigenomic stability.
Current projects in the lab include isolation of proximal and distal effectors of TORC1 and TORC2 and the use of genetically engineered yeast cells to screen for novel anti-TOR inhibitors.
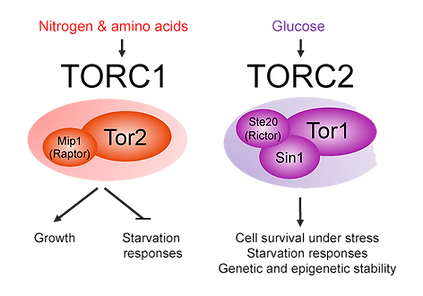